Enzyme Design and Evolution
In our group, we combine computational protein design with directed evolution to develop new enzymes. We have two main research goals: First, we want to gain a more fundamental understanding of how enzymes work and how their catalytic efficiency can be improved. On the other hand, we are working on the development of photoenzymes, i.e. light-activated biocatalysts that we want to use for the direct conversion of solar energy.
One focus of our research is biophotovoltaics - enzymes that can convert sunlight directly into electrical energy. Such systems could provide a sustainable alternative to conventional solar cells because they are based on biological materials, are potentially more environmentally friendly and can be more efficiently integrated into natural material cycles.
We are also developing photoenzymes that can efficiently convert nitrogen into ammonia. Ammonia is an essential component of fertilisers and is therefore central to global food production. However, the industrial production of ammonia is extremely energy-intensive, which we aim to avoid with our photoenzymes.
We leverage the strong expertise of surrounding groups in Marburg in the biocatalysis of nitrogen and CO₂ reduction, which complements our expertise in enzyme design and directed evolution. Our groups aim to design light-activated enzymes for carbon capture.
Novel Photoenzymes

Satisfying humankind's energy demand from sustainable sources is a critical challenge in the 21st century. To address this challenge, we employ design and evolution to create novel photo-biocatalysts by equipping existing enzymes with binding sites for affordable organic photosensitizers. We have recently created a photoenzyme with photovoltaic activity that we are currently incorporating into biohybrid solar cells. Our approach can be generally employed to induce photocatalytic activity – and we use it to create sustainable biocatalysts that target critical societal challenges such as renewable energies, carbon capture, food security, and solar fuels.
Creating Enzymes by Design and Evolution
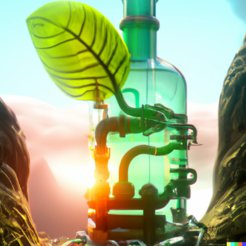
We combine computational design and directed evolution to create novel enzymes. Specifically, we use design and evolution to tailor artificial photocatalytic enzymes that harness solar energy for biocatalysis and energy production. Furthermore, we use experimental and computational methods to understand how enzymes work by dissecting how design and evolution give rise to catalytic activity.
Antimicrobrial Resistance
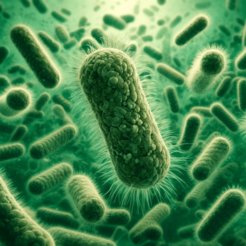
As antimicrobial resistance increases globally, infections with resistant bacteria pose a major public health threat. In our current work, we study the emergence of new resistances through the lens of directed evolution. Our work aims to aid the creation of novel antibiotics less prone to resistance and guide personalized health care.
How Enzymes Work
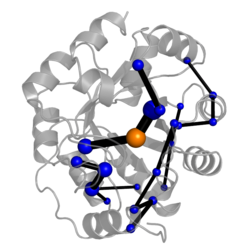
Enzymes can accelerate reactions with unequaled efficiency – understanding how enzymes work has been a longstanding goal in biochemistry. Comprehensive insights into the origins of catalysis will foster the creation of new biocatalysts for application. Directed evolution of designer enzymes can provide unparalleled insights into how enzymes work by revealing how catalytic activity emerges during evolution. To that end, we have created an enzyme accelerating its reaction by almost eight orders of magnitude using directed evolution. Evolution sometimes introduces new catalytic residues and complex catalytic motifs to boost catalysis. Notably, evolution not only introduces catalytic residues – but also stabilizes catalytically superior conformations to boots activity. These effects are not restricted to the active site. We showed that enzymes can recruit the whole protein for catalysis using dynamical networks that selectively recognize the transition state . Together, our insights suggest novel strategies to engineer more efficient enzymes for application.