Structure, function & regulation of the Type IVa pilus machine
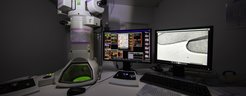
To move on surfaces bacteria use ubiquitous cell surface structures called Type IVa pili (T4aP), which are thin and flexible filaments of different lengths. T4aP-dependent motility depends on cycles of T4aP extension, surface adhesion, and retraction, which are driven by the T4aP machine.
Using a combination of genetics, cell biology, biochemistry, as well as transcriptome and proteome analyses we aim to explore the structure, function and regulation of the T4aPM in M. xanthus, which has emerged as an important model system to understand T4aPM biology.
Structure & function of the T4aP machine
The overall architecture of the unpiliated and piliated T4aP machine (T4aPM) of M. xanthus and the location of 15 conserved core proteins within this architecture has been elucidated as a large macromolecular complex that spans from the outer membrane across the periplasm and inner membrane (IM) to the cytoplasm (10.1126/science.aad2001, 10.1038/s41467-020-18803-z ). Our data led to the following working model:

Left: The unpiliated T4aP machine with the integral minor pilin/PilY1 complex essential for priming T4aP extension. Middle: The piliated T4aP machine in extension mode (PilB-extension ATPase bound at the base of the T4aPM) with minor pilin/PilY1 complex at the T4aP tip involved in adhesion to a surface. Right: The piliated T4aP machine in retraction mode (PilT-retraction ATPase bound at the base of the T4aPM) with minor pilin/PilY1 complex at the T4aP tip involved in stopping retraction when pulled back into the T4aPM. Left, middle, right: Minor pilins = FimU in yellow, PilV in orange, PilW in green, PilX in blue, OM = outer membrane, IM = inner membrane.
In the unpiliated T4aPM six of the15 proteins (PilQ, TsaP, PilP, PilO, PilN, PilM) are predicted to form ring-like structures in the entire cell envelope, while the platform protein PilC in the inner membrane is predicted to form a dimer or trimer. A complex containing four minor pilins (FimU, PilV, PilW, PilX) and a large PilY1 proteins is an integral part of the unpiliated T4aPM and is essential for priming T4aP extension.
T4aP extension occurs by binding of the hexameric PilB-extension ATPase to the base of the machine. Upon hydrolysis of ATP, PilB then rotates PilC. The rotating PilC protein scoops one subunit after the other of the major pilin PilA and adds it to the base of the growing T4a pilus. Upon T4aP extension the minor pilin/PilY1 complex becomes part of the T4aP at its tip, where it is predicted to be involved in adhesion.
The swap from PilB to PilT, and thus initiation of retraction, is predicted to be either a stochastic event or, to be induced by adhesion of the T4aP tip to the surface. In the latter model, adhesion of the T4aP tip causes conformational changes in the pilus that are communicated to the base of the T4aPM. These changes release PilB, and create a binding site for the hexameric PilT. Upon ATP hydrolysis PilT is then rotating PilC in the opposite direction so that the PilA subunits are removed from the base of the pilus and slip back into the inner membrane.
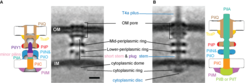
A: Component map (left) and subtomogram average (right) of the unpiliated M. xanthus T4aPM. Scale bar=10 nm. B: Subtomogram average (left) and component map (right) of the piliated M. xanthus T4aPM. A+B: Densities see in both subtomogram averages are shown in black, densities present only in the subtomogram average of the unpiliated (A) or piliated T4aPM (B) are shown in in colors. Minor pilins = FimU, PilV, PilW, PilX, OM = outer membrane, IM = inner membrane.
Comparison the subtomogram averages of the unpiliated and piliated T4aPM suggest dynamic conformational changes of the T4aPM components to occur upon T4aP formation, e.g. the diameter of the cytoplasmic ring increases, the OM pore undergoes conformational changes and the OM moves ~2 nm away from the mid-periplasmic ring. The cytoplasmic disc density, which is only seen in the subtomogram averages of the piliated T4aPM, is either formed by PilB or PilT, which bind to the base of the T4aPM in a mutually exclusive manner.
Currently we are trying to understand the underlying mechanism of the T4aPM, focusing on the components, their interactions, and their precise stoichiometry.
Structure & mechanical properties of the M. xanthus T4aP
Generally, T4aP are built from thousands of copies of the major pilin, which are usually not larger than 165 amino acids. In contrast, the major pilin PilA from M. xanthus is with 208 amino acid residues unusually large.We solved the structure of the M. xanthus T4aP at a resolution of 3.0 Å using cryo-EM on purified T4aP, which allowed us to build de novo an atomic model of the entire PilA. We learned that the size difference of PilA comes from its large C-terminal globular domain, and that the tight packing of this large globular domains creates a T4aP, which is more compact and rigid than any other T4aP yet described (10.1073/pnas.2321989121).
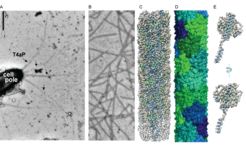
A: Image of a piliated M. xanthus cell by negative-staining. B: Image of purified T4aP by negative-staining. C: Cryo-EM structure of M. xanthus T4aP (8TJ2), with α-helical parts in light blue, β-strands in light green and less structured areas in grey. D: Structure of the M. xanthus T4aP showing the globular domains of PilA in different colors. E: Atomic model of the major pilin PilA, with α-helical parts in light blue, β-strands in light green and less structured areas in grey
We found that major T4a pilins can vary in size from ~40-300 amino acids, and that large pilins ≥166 amino acids are found in 13 out of 21 bacterial phyla. Interestingly, large major pilins are enriched in Betaproteobacteria, Cyanobacteria, Myxoccocota and Bdellovibrionota. We speculate that these large major T4a pilins enable the formation of compact & rigid T4aP, reflecting an adaptation to environmental conditions. Interestingly, Myxoccocota and Bdellovibrionota encode almost exclusively large major pilins.
Currently we are trying to understand what potential functional and environmental advantages might be associated with the formation of such compact and rigid T4aP.
Localization of the T4aPM & T4aP
In the rod-shaped bacterium M. xanthus T4aP are only extended at the leading cell pole, where upon extension and adhesion of T4aP, a force is created which pulls the cells in the direction of the adhered T4aP.Although the T4aPM is always present at both poles, it is only active at the leading cell pole. Consistent with this unipolar T4aP formation, the PilB extension ATPase almost exclusively localizes to the leading cell pole, while the PilT retraction ATPase localizes in a more bipolar asymmetric pattern and with the large cluster at the lagging cell pole.Therefore, at the lagging cell pole the T4aPM is only unpiliated, while at the leading cell pole the T4aPM can be either unpiliated, in the extension mode, or in the retraction mode.

A: The T4aPM core is present at both cell poles, but T4aP extension only occurs at the leading cell pole. PilB localizes almost exclusively at the leading cell pole, while PilT shows a bipolar asymmetric localization pattern with the larger cluster at the lagging cell pole. B: Polar localization of the six polarity proteins at the leading (piliated) and lagging cell pole (unpiliated). The bent arrows indicate exchange of GDP for GTP in MglA by RomR/RomX GEF activity (leading cell pole) and stimulation of GTPase activity of MglA by MglB/RomY (lagging cell pole). A+B: Size of the circles indicate the relative amount of a protein at a pole.
The activity of the T4aPM in M. xanthus is regulated by a so called polarity module, comprising at least 6 different proteins. The small Ras-like GTPase MglA, which is a nucleotide-dependent molecular switch that is inactive in the GDP-bound and active in the GTP-bound state, is the output of this module and localizes to and defines the leading cell pole. The remaining five proteins regulate the nucleotide-bound state and localization of MglA by acting as a guanine nucleotide exchange factor (GEF, RomR/RomX complex) or as a GTPase activating protein (GAP, MglB/RomY complex).
Occasionally M. xanthus cells reverse their direction of movement, during such a reversal movement of a cell first stops and then continues in the opposite direction. These reversals are induced by the Frz-chemosensory system, which senses a yet unknown signal. During a Frz-induced reversals, the old leading cell pole becomes the new lagging cell pole and vice versa. In parallel the polarity proteins, PilB, and PilT switch polarity and T4aP assemble at the new leading cell pole.
At the leading cell pole MglA interacts with additional proteins (SgmX & FrzS) to activate the T4aPM by stimulating polar localization of PilB. We recently identified the previously uncharacterized protein SopA as a fourth polar regulator involved in activating the T4aPM (10.1101/2024.03.11.584430v1).
Currently we are trying to understand how the Frz-system implements the polarity switch in M. xanthus and how activation of the T4aPM at the leading cell pole occurs on the molecular level.
Regulation of minor pilin/PilY1 gene clusters
M. xanthus encodes three sets of each four minor pilins and one PilY1 adhesion protein. For two of these three sets, i.e. those encoded by gene cluster_1 and gene cluster_3, experimental data support that they are each sufficient for T4aP-dependent motility and T4aP-formation under vegetative growth conditions. The PilY1 proteins encoded by the three gene clusters differ mostly by their different N-terminal domains, while their C-terminal PilY1 domains, supposed to be important for interaction with the minor pilins,are more conserved. Thanks to AlphaFold-Multimer we have high confidence models of the three priming complexes, in which the four minor pilins FimU, PilV, PilW and PilX are being sandwiched between PilA at the base and PilY1 at the top, and in which the N-terminal domains are at the ultimate tip of the complexes. We think that the different N-terminal domains of the PilY1 proteins have different functions in adhesion.
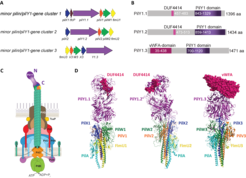
A: Cartoon of the three minor pilin/pilY1- gene clusters of M. xanthus. B: Cartoon of the domains of the three different PilY1 proteins. C: Cartoon of the piliated T4aPM with the minorpilin/PilY1-complex at the T4aP tip. The N-terminal domain of PilY1 is predicted to form the ultimate tip of the tip complex. D: AlphaFold-Multimer models of the three different minor pilin/PilY1-complexes and with their DUF4414 (PilY1.1 & PilY1.2) domains and the von Willebrand domain (vWFA, PilY1.3) shown as pink spheres.
Interestingly, experimental data are suggesting that the accumulation of cluster 1, 2 and 3 proteins is affected by environmental conditions, suggesting an underlying regulation determining which minor pilin/PilY1 complex is tipping the T4aP.
Altogether, the data and models suggest that the T4aPM of M. xanthus is composed of at least 15 proteins, which can be divided in ten core proteins (PilQ, TsaP, PilP, PilO, PilN, PilM, PilC, PilA, PilB or PilT) and five priming/tip complex proteins, encoded by either minor pilin/pilY1- gene cluster 1, cluster 2 or cluster 3.
Currently we are trying to understand the underlying mechanism determining which tip complex is tipping the T4aP.